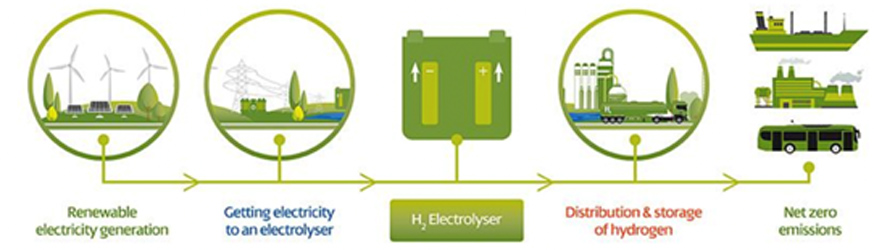
The energy demands have been raised drastically due to the rapid growth in the human population and its development activities. Electricity produced through renewable energy sources such as solar, onshore, and offshore wind and wave energy have succeeded remarkably over the past 20 years but the same cannot be used for other needy uses, in particular heat, heavy transport vehicles, and aviation. The non-renewable energy resources of petroleum and other co2 sources are increasing the pollution level and causing climate change. Innovative technologies and systems to be evolved to turn away the worst backwash of climate change. To bypass climate change, we need to implement comprehensive zero carbon-emission fuels as an alternative to the traditional breeds. Decarbonization is one of the earth-shattering complaints of the global economy. The main key to meet these complaints is forever expansion of renewable energy sources as well as the concept of sector coupling, meaning to integrate renewables in developed industry, energy, and mobility infrastructures with power to n-number of solutions using green hydrogen. Regardless of how it is produced, it has the same carbonless molecule. However, the ways it is produced vary widely, as do the emissions of greenhouse gases such as carbon dioxide (CO2) and methane (CH4). Hydrogen is emerging as one of the main options for energy storage from renewable energy sources with hydrogen-based fuels capable of carrying energy from renewable sources over long distances, from regions with abundant energy to energy-intensive regions thousands of kilometers away. There is unprecedented momentum around the world to fulfill hydrogen`s longstanding potential as a clean energy solution. The generation of hydrogen completely through the electrolysis process supported by the power generated from renewable energy sources is called ‘green hydrogen’. This method of obtaining green hydrogen could save 830 million tonnes of CO2 emitted annually when this gas is produced using fossil fuels according to the International Energy Agency. Green hydrogen will pay the way for the next frontier in clean energy as shown in Fig.1.
Fig.1 Green hydrogen production (Source: Scottish Power)
Green hydrogen has significantly lower carbon emissions than grey hydrogen, which is produced by steam reforming of natural gas. Green hydrogen could be a key catalyst in the global transition to a sustainable energy economy and net-zero emissions. The current challenges on green hydrogen production systems are reduced knowledge on optimum design, limited specialized workforce, high operational costs, and high energy losses. Apart from these challenges, Hydrogen will have a compiled benefit of having support in creeping change towards lower-carbon energy sources as it can be authored via natural gas and other non-renewable by-products. Inclusion with that, it can be frequented as an energy carrier; in other words, a medium to store energy from renewable and other sources. By looking forward to the real future, green hydrogen can be produced at scale with a zero-carbon footprint by using renewable sources such as solar or wind power inland/onshore to split water (electrolysis). But these resources are less abundant or seasonal onshore and the extremely abundant and auspicious source of renewable energy is the marine and hydrokinetic offshore energy resources such as waves, wind, and currents combinedly have 300 times more energy than the onshore energy that was currently consumed by humans. This shows that oceans are packed with a high volume of renewable energy which is untapped.
Fig.2 Green hydrogen through Ocean Renewable Energy Resources (Dr. Sameer )
As the dispatch of electricity produced by offshore energy resources is concerned, it is done in the form of electric current to onshore consumers through a convoluted transmission system. The power produced by wind turbines wave energy converters and tidal turbines are sent to the offshore substation through network cables bringing power from each energy source. More arguments arise as ocean energy grows in size and number as we move towards the deeper ocean to farther distances from the shore. To ship the entire power to the onshore substation, the produced power will be stepped up to the transmission level by the offshore substation. Based on current-carrying capacity, the size of the cable, locations of the substation, and investment in electrical systems is also a determinant of the cost of the offshore electrical infrastructure. Hence the dispatch of power from the offshore to the onshore environment had more significant issues involved in the dispatch. The conversion of offshore power to hydrogen is considered as one of the feasible alternatives, due to a sustainable demand for zero-carbon fuel. This can be made effective with the existing oil and gas infrastructure in the areas of empty gas reservoirs, platforms, and pipelines – can be opted for conversion, transport, and storage of hydrogen generated from offshore renewable energy.
The offshore energy-based hydrogen production projects have taken place in European Union with more efforts from UK and Scotland. One among them is Ocean Renewable Energy Fuel (EPSRC), an innovative £10M research project that investigates the potential of harnessing offshore wind and marine renewable energy to produce zero carbon-hydrogen and ammonia fuels targeting the conversion of offshore wind and marine renewable energy to liquid and gaseous fuels that leads net-zero carbon footprint. It is s a five-year project led by the University of Strathclyde, which also involves the Universities of Nottingham and Cardiff, Newcastle University, and Imperial College London. This shows the importance of green hydrogen in carbon footprint reduction. By carrying over the vast experience of onshore renewable resources, offshore renewable resources are noted to be the most progressive hydrogen production technology. Only a handful of works relate to wave hydrogen production, while research in tidal energy to hydrogen is almost negligible. One unique offshore hydrogen production concept presents a ship generating hydrogen while sailing. All studies have electrolyzers to produce hydrogen from water electrolysis. It is pertinent to mention here that most of these studies do not specify the storage of produced hydrogen gas and its subsequent transmission from offshore platform/facility to the onshore consumers. Storage and distribution of the energy vector (hydrogen in this case) in whichever form (gas/liquid) are two equally significant elements of any proposed future supply chain. The challenge given the large-scale introduction of offshore renewable energies for green hydrogen production lies in the coupling of electrolyzers with offshore energy resources. Other aspects in offshore electrolysis include the sizing of the systems, development of platforms, and storage of the generated hydrogen that should be focused on shortly for the efficient net-zero carbon environment.
Nickel-based superalloys are yet another group of materials that have admirable mechanical properties in comparison with stainless steel at temperatures as high as 700°C and can achieve a yield strength that is about 3 times higher. Given their high strength at elevated temperatures, they are increasingly considered for such aircraft engine parts like combustor and turbine sections where the operating temperatures are easily in the range of 1100 and 1250°C.
Despite all the above advantages, the percentage of the alloys used in an aircraft today is on a slight decline with the advent of newer materials especially composite materials. Composite materials are a new class of advanced materials that have revolutionized the engineering materials domain with their exceptional properties. The aerospace sector is heavily inclined towards the usage of composite materials majorly due to their higher specific strength (strength/density) combined with better fatigue and corrosion resistance than that offered by most metal alloys. For instance, carbon fiber reinforced polymer composite materials offer good strength with very low density. The density of these materials is just one-fifth that of steel and three-fifth of aluminum alloys. Ceramic-based composite materials are proved to sustain higher operating temperatures of up to 1400°C which can easily cater to the increasing demand for aircraft speed. Carbon fiber reinforced silicon carbide composite materials are candidate materials for aircraft brakes where temperatures encountered can go up to 1200°C in case of emergency braking situations. The aerospace industry is increasingly considering composite materials for not just structural applications but also some engine parts. Composite materials today contribute to around 25% of the Airbus A380 and 50% of the Boeing 787 aircraft which is quite remarkable and needless to say it is only going to get better with time.
Dr. Manmathakrishnan P., Assistant Professor, Alliance College of Engineering and Design
Prof. Vijayalakshmi Bharathi, Assistant Professor, Alliance College of Engineering and Design