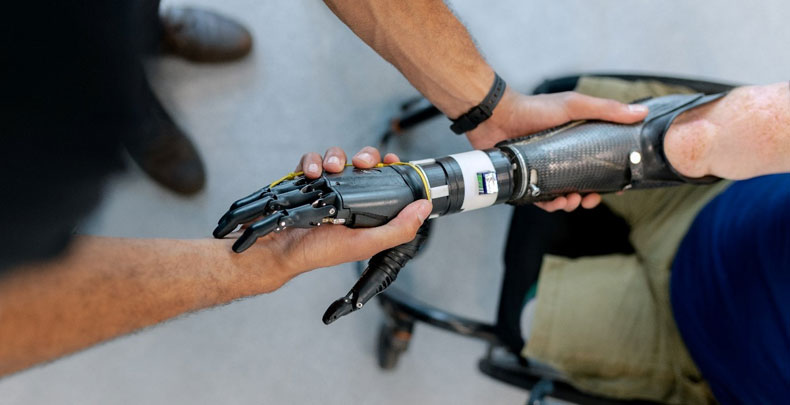
The Human body is an engineering marvel. One associates the functioning and treatment of the human body as a domain of medical and pharmaceutical experts. However, various fields of engineering are associated with the “running and maintenance” of the human body. The skeletal structure comes within the domain of mechanical and civil engineers. Bones take up shapes and dimensions based on applied loads from day-to-day activities. The pumping of the heart, inhalation, and exhalation of air, filtering within the kidneys, fluid flow, and movement at the joints come under the domain of mechanical engineering. The functioning of the nervous system that controls various functions of the body can be related to the domain of electrical and electronics engineering. All support equipment, from X-ray machines to surgical instruments are designed and manufactured by engineers. Joint and limb prostheses, artificial heart valves, pacemakers, catheters, dialysis machines, heart-lung machines, and syringes are some of the numerous pieces of equipment utilized by medical professionals, which require engineers for their creation. The performance of a sportsperson and the fitness of an individual can be enhanced by careful studies and regimens developed by engineers. Therefore, it can be said that “A physician cannot function without the support of engineers”. Materials that come in contact with the body (externally or internally) need to be biocompatible and undergo a series of tests, before being commercialized. They are therefore termed as “biomaterials”.
According to experts, a biomaterial is defined as “A material intended to interface with biological systems to evaluate, treat, augment, or replace any tissue, organ or function of the body”. Therefore, the interaction of a biomaterial with its environment is of prime importance. The term “biocompatibility” is defined as “The ability of a material to perform with an appropriate host response in a specific application”. Therefore, a bio materialist is responsible for alterations to the composition of a biomaterial and/or its fabrication process to control the biological response and produce an implant with maximal biocompatibility.
All the broad classes of materials (i.e., metals, ceramics, and polymers) are used as biomaterials due to the unique advantages of each group. Metals are strong and relatively easily formed into complex shapes. Examples of metals used as biomaterials are cobalt-chromium alloys, Titanium alloys, gold, stainless steel, and silver-tin-copper alloys. Examples of ceramic biomaterials are Alumina, Zirconia, Bioactive glasses, and Calcium Phosphates. Examples of polymeric biomaterials are polyhydroxy methacrylate, polyethylene, polyethylene glycol, polymethyl methacrylate. Some naturally derived polymers used as biomaterials are Alginate, Chitosan, Collagen, Elastin, Fibrin.
Although the wide use of biomaterials is relatively new (less than 100 years), evidence of the use of metal dental implants as early as 200A.D. has been found. Linen sutures were used by the early Egyptians. Catgut was used in the Middle Ages in Europe. In South Africa and India, the heads of large, biting ants clamped wound edges together. Galen of Pergamon (130–200 AD) described ligatures of gold wire. In 1816, Philip Physick, University of Pennsylvania Professor of Surgery, suggested the use of lead wire sutures, noting little reaction. J. Marion Sims of Alabama had a jeweller fabricate sutures of silver wire, and in 1849 performed many successful operations with this metal.
The two decades after World War II saw the advent of the first successful hip joint implant (metallic), kidney dialysis machines (originally made of cellulose), and vascular grafts (made of silk). As of today, a few examples of the applications of biomaterials are in artificial heart valves, contact lenses, cardio-vascular devices, orthopaedic-musculoskeletal devices, pacemakers, blood bags, catheters, heart-lung machines, coronary stents, intraocular lenses, and more. Initially, biomaterials were designed to be inert (no reaction with the body), thereby decreasing the potential for a negative response to the implant. Later, certain biomaterials were designed to be bio-active, interacting with tissue positively to promote localized healing.
The shape and size of the implant, its localization in the body, and the chemical, physical and mechanical bulk and surface properties of the biomaterial affect its degradation in vivo (within the living being). Although the temperature and pH of body fluids are relatively mild, during inflammation, cells may be recruited to the area and produce active substances to transform the region into a harsh environment, promoting material degradation. Depending on the implant site and the nature of the biomaterial, other reactions may also ensue, such as activation of the immune system, localized blood clotting, infection, tumour formation, or implant calcification. Some of these reactions may be beneficial. For example, calcification of an implant used to support bone tissue may be necessary to ensure good integration between the biomaterial and the surrounding bone.
Biomechanics is the study of human movement and the forces acting upon it both internal and external, during motion and when stationary. It is important to study because it helps to identify the best techniques to act. It allows a skill to be broken down into its sub-skills. For example, a tennis player serving can break down the entire service into sub-tasks and refine them to get the most efficient and effective service possible. The study of biomechanics related to sports is known by the term “Kinesiology”. Some of the most highly ranked universities in the world offer Kinesiology as a major discipline of study. The efficient motion of limbs and the development of the tissues involved are vital to enhanced sports performance. Some of the tissues involved in limb motion are bones, cartilage, ligaments, and tendons. Ligaments connect one bone to another, tendons connect muscle to bone, while cartilage resides at the ends of bones, providing a cushioning effect and a low coefficient of friction. The strength and functioning of these tissues decide the level to which limb motion can take place. Proper signalling from the brain and nervous system also affects body movement. Therefore, deficiencies in human locomotion, for example, can indicate improper cognitive abilities. Other popular areas within the field of biomechanics are the cardio-vascular system, cardio-pulmonary system, digestion and peristalsis, and kidney filtration.
The heart along with blood vessels makes up the cardiovascular system. Its functions are to transport important substances such as oxygen and nutrients between tissues and organs, helps transport and eliminate waste products, these materials are carried by the blood through blood vessels and are moved by the pumping action of the heart, there are two main pathways-the pulmonary circuits from the heart to the lungs and back and the systemic circuit between the heart and all other areas of the body. The circulation system contains approximately 100,000 km of blood vessels and it pumps approximately 7.5 kilolitres of blood per day, for an adult. The volume of blood in an adult is around 5 litres. The density of human blood is approximately 1050kg/m3. The heart is a four-chambered, hollow muscular organ approximately the size of the person’s fist. Functions of the heart are generating adequate blood pressure, routing blood, separates pulmonary and systemic circulations, ensures one-way blood flow, and regulates blood supply based on metabolic needs.
The locomotion cycle is known as the “Gait cycle”. It consists of the swing phase (when the foot is off the ground) and the stance phase (when the foot is in contact with the ground). These phases are subdivided into smaller phases. Gait labs are equipped with cameras, force platforms, and reflectors to analyse a person’s gait. A limb prosthesis can be defined as “An artificial replacement for any or all parts of the lower or upper extremities, it is a device that is designed to replace, as much as possible, the function and/or appearance of a missing limb or body part”. A limb prosthesis is used to provide an individual who has an amputated limb with the opportunity to perform functional tasks, which may not be possible without the limb. The prosthesis may also be made for use during activities or sports, such as dancing, swimming, cycling, golfing, and climbing. A joint prosthesis is provided to replace a bone joint that has degraded or damaged cartilage. Cartilage tissue does not have an inherent healing capacity due to the lack of blood supply.
A medical device can be classified as Class I – low risk (crutches, bedpans, adhesive bandages), Class II – Moderate risk (catheters, needles, contact lenses), or Class III – high risk (pacemakers, coronary stents, joint replacements). They need to go through a series of testing before they can be commercialized. The stages for high-risk devices are in-vitro (within a lab) cell culture testing, in-vivo (within a living being) animal studies, ex-vivo (within human tissue or organs outside the body) human studies, and within human studies known as “clinical trials”.
One of the current areas of medical advancement is Tissue Engineering defined as” The application of principles and methods of engineering and life sciences toward the fundamental understanding of structure-function relationships in normal and pathological mammalian tissues and the development of biological substitutes to restore, maintain or improve tissue function.” It is the study of growing tissue in a lab, called ”Tissue culture”.
The components of tissue culture are:
- Cells are organized into functional units
- The extracellular matrix
- Scaffolding architecture
The scaffolding provides structure to the tissue and is usually made of synthetic or natural polymers.
Dr. Rahul Ribeiro
Associate Professor
Alliance College of Engineering and Design