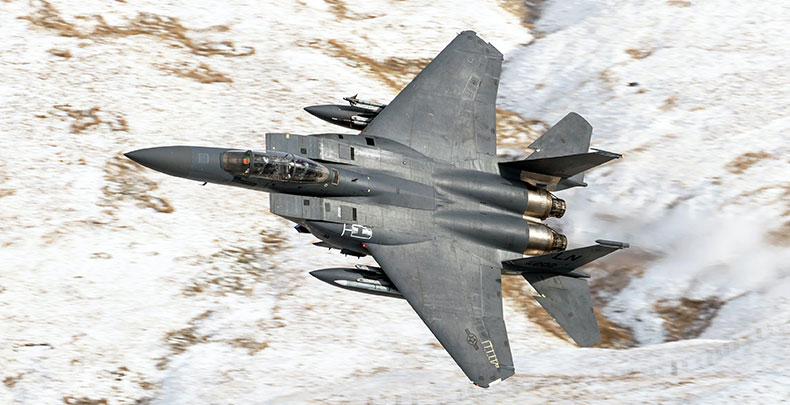
The emergence of Computational Fluid Dynamics in the last 60 years is fantastic, especially in providing a complete low-cost technical solution to the Aerospace and Defence sectors, compared to the previous experimental and numerical techniques. Starting from the formulation of the governing equations (potential flow, Euler, RANS), the combination of advances in computer power and algorithms, Riemann-based schemes for gas dynamics (Godunov), 2nd-order dissipative schemes for hyperbolic equations (Lax-Wendroff), efficient explicit methods for Navier-Stokes (MacCormack), panel method (Hess-Smith), type-dependent differencing (Murman-Cole), complex characteristics (Garabedian), rotated difference (Jameson), multigrids (Brandt), flux splitting (Steger-Warming), shock capturing via controlled diffusion (Jameson-Schmit-Turkel), approximate Riemann solver (Roe), total variation diminishing (Harten), multigrids (Jameson, Ni), and up to the solution of a complete airplane (Jameson-Baker-Weatherill) are the milestones of CFD.
Since CFD is a numerical technique, especially for dealing the applications in Aerospace and Defence, there is a challenge in computing technique. Let us have a quick look into the development of computers in 30 years, 1970-CDC6600 (birth of supercomputer)-1 Megaflops, 1980-Cray 1 Vector Computer-100 Megaflops, 1994-IBM SP2 Parallel Computer-10 Gigaflops, 2007-Linux Clusters-100 Teraflops, 2007-Box Cluster Four 3 GHz dual-core CPUs (24 Gigaflops peak)- 2.5 Gigaflops, 2009-HP Pavilion Quad-core Notebook-1 Gigaflops, 2011-MacBook Pro Quad-core Laptop-2.5 Gigaflops, etc., where Flops – Floating point operations per second.
A typical CFD analysis begins at problem identification with defining goals and identifying the domain, pre-processing with geometry, mesh, physics, solver settings, solving the problem with computing solution, and finally ending with simulation to examine and interpret the results.
The CFD for Aerospace and Defence will provide solutions in the areas such as aerodynamics (Fluid Analysis), structure-fluid interface analysis, heat transfer analysis, etc., Here the heat transfer analysis is done not only in engines (propulsion) but also in the components such as de-icing unit, heat in avionics system, heat in electrical and electronics systems, heat in landing gear wheels, air-conditioning unit, fuselage and cockpit pressurization units, etc.
Since the association of CFD with fluid analysis, a discussion about Aerodynamics, Aerodynamic analysis in Aircraft comprises pressure, velocity, lift, drag, etc., at the significant points. Before CFD, analysis was done by some other numerical techniques and was especially carried out with the help of wind tunnels. The Wind Tunnel is a device where the prototype of the object will be tested or the original object will be mounted (static) in the test section and the flying environment will be created inside the tunnel. With the help of sensors, the above said required parameters could be experimentally measured.
How is CFD better than the experimental technique (wind tunnel)?
The prime advantage is the fact that the CFD is a whole-field technique (comprehensive information). That is, all the above-said properties can be found in each point of the tested object. Moreover, it is cost-wise cheap; time taken is less (speed); suitable for any scale; repeatable; from the security point of view safe; and has ability to simulate actual conditions.
The CFD contribution to the periodical technology developments in Airbus and Boeing aircraft is extraordinary. The design of high-speed wing, wingtips, vortex generators, high lifting devices, wing-body fairing, environmental control system inlet, engine-airframe integration, nacelle, control surfaces failure analysis, flutter, vertical tail & aft body, avionics cooling, stability & control, APU inlet & ducting, exhaust system, engine bay thermal analysis, APU & propulsion fire suppression, cabin ventilation, cabin noise, ice prediction, belly fairing, nozzle, fuel system, flow control devices (VG/Strakes), engine core compartment, pack bay thermal analysis, performance prediction, etc., are some of the parameters completely streamlined and improvised both in Airbus and Boeing with the help of CFD.
So, after CFD, the utility of a wind tunnel for testing the aircraft is drastically reduced. Especially in the growth and development of Boeing, the contribution of wind tunnel testing was reduced from 77 during the 1980-s to 11 during 2005. Technically and economically, it was a viable option. A502, A488, TRANAIR, TLNS3D, TRANAIR optimization, TLNS3D-MB/ZEUS, CFL3D/ZEUS overflow CFD++, and Unstructured adaptive grid 3-D N-S were some of the Boeing CFD tools utilized.
Let us discuss one case study. In 20 years, from 1955 to 1975, wind-tunnel-based development indicated nacelles could not be placed too close to the wing without excessive drag. Then, within the next two years, 1975 to 1977, joint CFD/Wind Tunnel studies unlocked the secret of nacelle/wing interference drag. The interference drag is the net effect and impact in drag of any integrated parts design. Around 1980, 707/CFM56 design and flight test validated CFD concepts. Now, it was about the impact of CFD on a Boeing series B737-300 program. “Without the understanding gained from CFD, there would not have been a 737-300 program!”, was the statement of Walt Gillette, Manager, 737 Aerodynamics – then Vice President, 787 Engineering.
Some modifications in the B737-300 program from the previous versions with the help of CFD were the success of that aircraft, as exclaimed by Mr. Walt Gillette. The improvisation of design promoted the sales of B737-300. Now the case study. In the early 1980s, CFM56-3 was selected by Boeing to exclusively power the latest Boeing 737 variant, the 737-300. In Boeing 737, several modifications to the engine were required as the wings were closer to the ground than previous applications of the CFM56. The fan’s diameter was reduced, which resulted in decreased bypass ratio, along with the movement of the engine accessory gearbox from the bottom of the engine to the side of the engine, which gave the engine nacelle its distinctive flat-bottomed shape. The reduction of the bypass ratio caused a reduction in overall thrust, from 24,000 to 20,000 lbf (107 to 89 kN). Another significant change was the attachment of the engine with wing, without pylon. Because of all these changes in design, the interference drag was very much minimized to improve forward thrust. This was the success of the Boeing 737-300 with the technology of CFD.
Now a brief about CFD in Aero-Engines. CFD modeling needs to be specifically addressed to Compressor – RANS / URANS / LES; to Combustion Chamber – RANS/LES; to Turbine – RANS / URANS / LES; to Afterburner – RANS/LES, where RANS is Reynolds-averaged Navier–Stokes equations; URANS – Unsteady Reynolds-averaged Navier–Stokes equations; and LES – Large Eddy simulation. Each component has distinct flow physics such as Flow separation and Transition, Multi-phase Flows, Chemical Reaction, Mixing, Cooling, Heat Transfer, etc. Turbulence modeling is required since most flows in practice are turbulent, and a very fine grid necessary to describe all length scales. It is a fact that most engineering combustion systems involve turbulent flames. The bottleneck of CFD combustion solvers is the modeling of turbulence – chemistry interaction. Some of the chemical reactions modeling approaches are the Eddy-Break-up model, Flamelet model, and detailed chemistry. Modern CFD codes for the formulation of field equations using the Eulerian approach are Fluent, CFD, CFD++, CFD-ACE+, etc.
Some of the applications of CFD for Helicopter design and development are:
- Several internal and external flow concerns of the Helicopter and its systems
- Optimization of Fuselage shape, Engine Cowling, Tail-boom, Empennage, etc.
- Undesired flow interactions investigation and adequate solutions identification for the concerns present
- Rotor-Fuselage flow interactions. Estimation of Forces & Moments of Helicopter & Systems
- Engine and Exhaust bay cooling studies
- Missile separation trajectory estimation
- Engine Air Intake and Exhaust Systems Integration study
- Main and Tail Rotors Design & Optimization
- Fuel tanks filling and associated events study
- Optimization of external appendages for Drag reduction and adequate stability
The challenges of CFD in Aerospace and Defence are:
- Convergence-related issues of higher-order schemes and turbulence models.
- Prediction of transition.
- Hexahedral blocking strategy for models having fillets, slots and cooling holes.
- Automatic Re-meshing (Mesh Morphing).
- Interfacing CFD and Structural Codes: Flutter Analysis.
- Turbulent-Combustion Interaction model.
- Prediction of Soot and NOx.
- Prediction of Ignition through LES.
The future of CFD in Aerospace and Defence will be based on:
- By improving base research and technology (R/T) development program for simulation-based analysis and design technologies.
- Developing and maintaining an integrated simulation and software development infrastructure to enable rapid CFD technology maturation.
- Developing and improving algorithms, hardware, and unstructured hybrid grids.
- Utilizing HPC systems for large-scale CFD development and testing.
- Developing and executing integrated experimental testing and computational validation campaigns.
- Developing faster, and leverage improved collaborations with key research partners and industry stakeholders across disciplines within the broader scientific and engineering communities.
Prof. K. Velmurugarajan
Head / Department of Aerospace Engineering
Alliance College of Engineering and Design